Civil GPS Jammer Signal Traits: Key Insights
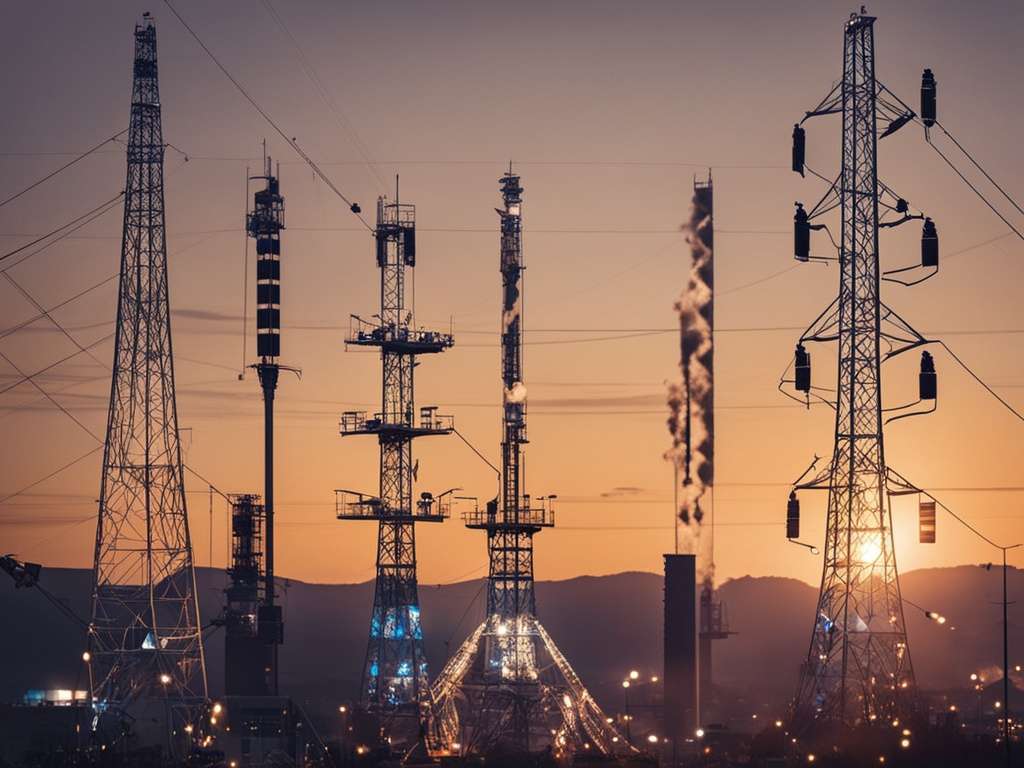
The rise of GPS integration into civilian infrastructure has led to a corresponding surge in the system’s susceptibility to jamming and interference. This vulnerability is exploited by lawbreakers seeking to disrupt GPS-enabled systems, leading to the widespread availability of GPS jamming devices online. These affordable devices, often priced lower than basic GPS receivers, pose a considerable threat to the seamless operation of numerous GPS-reliant systems. The landscape of intentional radio frequency (RF) interference is diverse, encompassing various forms such as tones, swept waveforms, pulses, narrowband noise, and broadband noise, all capable of disrupting GPS signals.
Mitigating the effects of jamming and interference, as well as locating their sources, can be achieved through various methods. These methods can be further enhanced by utilizing prior information about the interference source. This article provides such prior information, focusing on a set of jammers and assessing their associated threats. Our findings are based on two tests. The first test involved recording raw RF data from selected jammers and analyzing it using fast Fourier transform (FFT) spectral methods. The second test evaluated the effective range of a subset of gps jammers using a commercial off-the-shelf (COTS) receiver. The results presented in this article are based on 18 civil GPS jammers. However, it’s important to note that there are other types of GPS jammers available for sale that were not tested. Additionally, the behavior and design of civil jammers are likely to evolve over time.
Civil gps jammer devices, claiming to jam or “block” GPS signals, are easily accessible through various websites and online entities. The cost of these devices varies significantly, ranging from just a few tens of dollars to several hundred. However, their price does not seem to correlate with the features and effectiveness claimed by the suppliers. Advertised effective ranges span from a few meters to several tens of meters, but the actual effective ranges are often much greater. Additionally, the claimed and true power consumptions range widely, from a fraction of a watt to several watts. In this article, we draw conclusions based solely on the jammers that we tested.
We examined various GPS jammers and categorized them into three distinct groups based on their morphology. These jammers, designed to plug into an automotive 12-volt auxiliary power supply outlet (cigarette lighter socket), constitute Group 1. Another set, Group 2, includes jammers powered by an internal rechargeable battery and featuring an external antenna connected via an SMA connector. Lastly, Group 3 comprises jammers disguised as cell phones; they have batteries but no external antennas. Figure 1 illustrates an example from each of these three groups. Across all 18 jammers, most broadcast power near the L1 carrier frequency, six broadcast power near the L2 carrier frequency, and none broadcast power near the L5 carrier frequency.
In this article, we focus on the power levels broadcast within the GPS L1 and L2 bands by various signal jammers. Our findings reveal that many of these devices possess the potential for easy modification, enabling them to broadcast significantly higher power within the GPS bands. Notably, while some jammers also operate at frequencies outside the GPS spectrum, such as cellular phone or Wi-Fi bands, these are not the subject of our discussion. During our testing, we removed the jammer antennas to ensure consistent measurements. However, it’s important to recognize that in real-world applications, the antennas play a crucial role in shaping the jammer’s behavior. Specifically, Group 1 and Group 2 jammers utilize loaded monopole antennas, whereas Group 3 jammers rely on electrically short helical antennas, which exhibit a gain pattern similar to that of the loaded monopoles.
These antennas emit linearly polarized radiation, unlike the right-hand circular polarization of GPS signals. Due to this polarization mismatch, right-hand circularly polarized GPS receiver antennas experience a power loss. This innovative technology ensures a smooth signal flow while maintaining optimal reception quality, despite the inherent power reduction caused by the polarization difference.
- How Effective Are Jammer Signals? A Characteristics Test
- How Effective Are Signal Jammers? Test Results Revealed
How Effective Are Jammer Signals? A Characteristics Test
The core objective of our initial test series was to capture intricate samples of jamming signals and deduce the jammer’s characteristics from these datasets. We employed a two-phase approach for effective data collection. Initially, a spectrum analyzer was utilized to pinpoint the frequency range of the jamming signal near L1 and L2. Following this, the identified frequency details were used to set the center frequency of a multipurpose RF digitization and signal storage device, equipped with a 12-drive RAID storage array. Subsequent offline analyses were conducted on the recorded data. Our testing procedure involved the following steps: For the first two groups, the jammer was positioned within an RF-shielded test enclosure (refer to FIGURE 2) to eliminate any signal leakage. Its SMA signal output port was then connected to the relevant data collection device using a shielded coaxial cable.
The RF enclosure was primarily used as a precautionary measure, ensuring that signals passed from the inside to the outside through the built-in coaxial feed-through. It’s noteworthy that for Group 1 and 2 jammers, no jammer signal radiation occurred even within the enclosure. On the other hand, Group 3 jammers, lacking external antennas, were permitted to radiate within the RF enclosure using their internal antennas. To capture these signals, an actively amplified receiving patch antenna was positioned inside the RF enclosure. This antenna’s output was then connected to the relevant RF recording device via the enclosure’s coaxial feed-through. The jammer and receiving antenna were separated by approximately 14 centimeters, with the patch antenna’s field-of-view center pointed directly at the jammer. The jammer was oriented such that the axis of its helical antenna was perpendicular to the line connecting the receiving antenna and the jammer.
How Effective Are Signal Jammers? Test Results Revealed
Through FFT spectral methods and measurements of in-band power, we analyzed the signals of 18 jammers, although only a representative subset is discussed here. Figure 3 illustrates the results of this analysis for a typical jammer from Group 1. This figure’s top plot graphs frequency on the vertical scale against time on the horizontal scale, while the bottom plot graphs power on the vertical scale versus time on the horizontal scale. Each vertical slice of the recorded RF data plot represents a single FFT frequency spectrum, covering 62.5 MHz centered on the L1 band with a resolution of approximately 1 MHz. The relative power spectral density of each slice is indicated by color. Both plots’ time axes span 80 microseconds. The upper plot of Figure 3 clearly shows a linear frequency modulation interspersed with rapid resets – a series of linear chirps. Each sweep takes nine microseconds and spans a range of about 14 MHz.
Exploring jammer signal properties, we observed notable variations within Group 1 devices. Despite their seemingly identical exteriors, these jammers exhibited distinct signal behaviors, differing in frequency modulation and power output within the L1 GPS band. This civilian frequency range, marked by a horizontal red line in our top plot, revealed a consistent power output of approximately 20 milliwatts across the devices. Intriguingly, three of these jammers seemed to share a common model, while one stood apart. All exclusively broadcast at L1. Furthermore, a unique jammer from Group 2 caught our attention due to its dual distinctions, as visualized in FIGURE 4. This device specifically targeted the L2 spectrum, with its center frequency denoted by the red line, presenting an unusual spectral profile compared to its counterparts.
Discover the distinct features of advanced signal jammers. Unlike Figure 3, the jammer showcased in Figure 4 exhibits a triangular wave frequency modulation, standing out with its remarkably high modulation frequency. This rapid modulation boasts a period of merely 1 microsecond, a tenfold decrease compared to its counterparts. Moreover, its horizontal scale spans just 8 microseconds, offering a closer look at its functionalities. While Group 2 jammers typically utilize sawtooth frequency modulations as seen in Figure 3, this particular model goes beyond, broadcasting jamming power at both L1 and L2 frequencies.å¼å¾æ³¨æçæ¯,åªæå¦ä¸ä¸ªGroup 2å¹²æ°å¨å
·å¤L2å¹²æ°è½åãAlthough two jammers fell short due to their poorly designed L1 frequency modulation, with no jamming power nearer than 4.6 MHz from the L1 carrier, this jammer excels in its range and versatility. Furthermore, Group 3 jammers introduce another unusual modulation, with L1 results captured in FIGURE 5, showcasing the diverse capabilities of these devices.
Four jammers in Group 3 broadcast power at L1, L2, and additional frequency bands, typically utilizing a standard sawtooth frequency modulation. However, as seen in the upper plot of the figure, this modulation seems to be distorted by sudden frequency jumps, resulting in a linear-type frequency modulation. Despite its irregular nature, this waveform maintains its jamming efficacy. Three of the jammers appeared to be of the same model, while a fourth differed. Figure 5 represents an exception, where additional types of distortion from the nominal sawtooth frequency modulation have been observed in some jammers. For brevity, discussion of each additional variation has been omitted here. For more details, refer to the authors’ companion conference paper listed in the Further Reading sidebar.